9.1. Insects of litter and soil
Litter is fallen vegetative debris, comprising materials such as leaves, twigs, wood, fruit, and flowers in various states of decay. The processes that lead to the incorporation of recently fallen vegetation into the humus layer of the soil involve degradation by micro-organisms, such as bacteria, protists, and fungi. The actions of nematodes, earthworms, and terrestrial arthropods, including crustaceans, mites, and a range of hexapods (Fig. 9.1), mechanically break down large particles and deposit finer particles as feces. Acari (mites), termites (Isoptera), ants (Formicidae), and many beetles (Coleoptera) are important arthropods of litter and humus-rich soils. The immature stages of many insects, including beetles, flies (Diptera), and moths (Lepidoptera), may be abundant in litter and soils. For example, in Australian forests and woodlands, the eucalypt leaf litter is consumed by larvae of many oecophorid moths and certain chrysomelid leaf beetles. The soil fauna also includes many non- insect hexapods (Collembola, Protura, and Diplura) and primitively wingless insects, the Archaeognatha and Zygentoma. Many Blattodea, Orthoptera, and Dermaptera occur only in terrestrial litter — a habitat to which several of the minor orders of insects, the Zoraptera, Embiidina, and Grylloblattodea, are restricted. Soils that are permanently or regularly waterlogged, such as marshes and riparian (stream marginal) habitats, intergrade into the fully aquatic habitats described in Chapter 10 and show faunal similarities.
In a soil profile, the transition from the upper, recently fallen litter to the lower well-decomposed litter to the humus-rich soil below may be gradual. Certain arthropods may be confined to a particular layer or depth and show a distinct behavior and morphology appropriate to the depth. For example, amongst the Collembola, Onychurus lives in deep soil layers and has reduced appendages, is blind and white, and lacks a furcula, the characteristic collembolan springing organ. At intermediate soil depths, Hypogastrura has simple eyes, and short appendages with the furcula shorter than half the body length. In contrast, Collembola such as Orchesella that live amongst the superficial leaf litter have larger eyes, longer appendages, and an elongate furcula, more than half as long as the body.
A suite of morphological variations can be seen in soil insects. Larvae often have well-developed legs to permit active movement through the soil, and pupae frequently have spinose transverse bands that assist movement to the soil surface for eclosion. Many adult soil-dwelling insects have reduced eyes and their wings are protected by hardened fore wings, or are reduced (brachypterous), or lost altogether (apterous) or, as in the reproductives of ants and termites, shed after the dispersal flight (deciduous, or caducous). Flightlessness (that is either through primary absence or secondary loss of wings) in ground-dwelling organisms may be countered by jumping as a means of evading predation: the collembolan furcula is a spring mechanism and the alticine Coleoptera (“flea-beetles”) and terrestrial Orthoptera can leap to safety. However, jumping is of little value in subterranean organisms. In these insects, the fore legs may be modified for digging (Fig. 9.2) as fossorial limbs, seen in groups that construct tunnels, such as mole crickets (as depicted in the vignette of this chapter), immature cicadas, and many beetles.
The distribution of subterranean insects changes seasonally. The constant temperatures at greater soil depths are attractive in winter as a means of avoiding low temperatures above ground. The level of water in the soil is important in governing both vertical and horizontal distributions. Frequently, larvae of subterranean insects that live in moist soils will seek drier sites for pupation, perhaps to reduce the risks of fungal disease during the immobile pupal stage. The subterranean nests of ants usually are located in drier areas, or the nest entrance is elevated above the soil surface to prevent flooding during rain, or the whole nest may be elevated to avoid excess ground moisture. Location and design of the nests of ants and termites is very important to the regulation of humidity and temperature because, unlike social wasps and bees, they cannot ventilate their nests by fanning, although they can migrate within nests or, in some species, between them. The passive regulation of the internal nest environment is exemplified by termites of Amitermes (see Fig. 12.9) and Macrotermes (see Fig. 12.10), which maintain an internal environment suitable for the growth of particular fungi that serve as food (section 12.2.4).
Many soil-dwelling hexapods derive their nutrition from ingesting large volumes of soil containing dead and decaying vegetable and animal debris and associated microorganisms. These bulk-feeders, known as saprophages or detritivores, include hexapods such as some Collembola, beetle larvae, and certain termites (Isoptera: Termitinae, including Termes and relatives). Although these have not been demonstrated to possess symbiotic gut protists they appear able to digest cellulose from the humus layers of the soil. Copious excreta (feces) is produced and these organisms clearly play a significant role in structuring soils of the tropics and subtropics.
For arthropods that consume humic soils, the subsoil parts of plants (the roots) will be encountered frequently. The fine parts of roots often have particular associations with fungal mycorrhizae and rhizobacteria, forming a zone called the rhizosphere. Bacterial and fungal densities are an order of magnitude higher in soil close to the rhizosphere compared with soil distant from roots, and microarthropod densities are correspondingly higher close to the rhizosphere. The selective grazing of Collembola, for example, can curtail growth of fungi that are pathogenic to plants, and their movements aid in transport of beneficial fungi and bacteria to the rhizosphere. Furthermore, interactions between microarthropods and fungi in the rhizosphere and elsewhere may aid in mineralization of nitrogen and phosphates, making these elements available to plants; but further experimental evidence is required to quantify these beneficial roles.
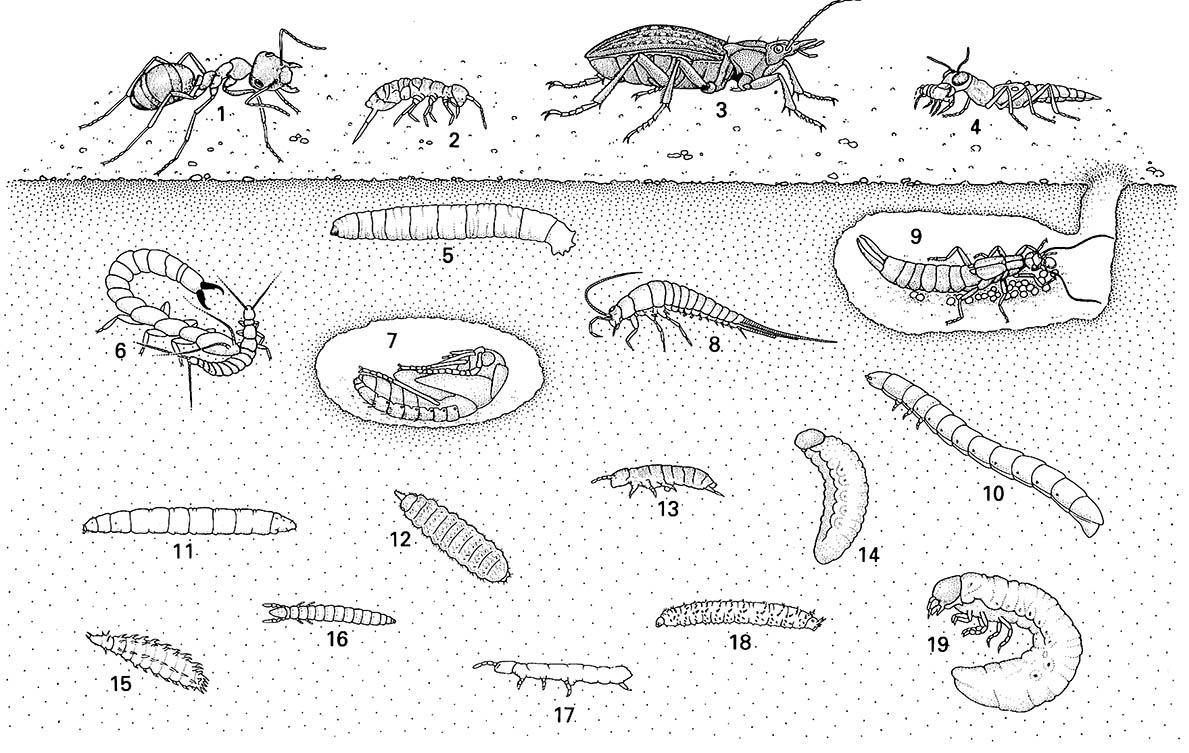
Note that organisms living on the soil surface and in litter have longer legs than those found deeper in the ground. Organisms occurring deep in th e soil usually are legless or have reduced legs; they are unpigmented and often blind. The organisms depicted are: (1) worker of a woo d ant (Hymenoptera: Formicidae); (2) springtail (Collembola: Isotomidae); (3) ground beetle (Coleoptera: Carabidae); (4) rove beetle (Coleoptera: Staphylinidae) eating a springtail; (5) larva of a crane fly (Diptera: Tipulidae); (6) japygid dipluran (Dip lura: Japygidae) attacking a smaller campodeid dipluran; (7) pupa of a ground beetle (Coleoptera: Carabidae); (8) bristletail (Archaeognatha: Machilidae); (9) female earwig (Dermaptera: Labiduridae) tending her eggs; (10) wireworm, larva of a tenebrionid beetle (Coleoptera: Tenebrionidae); (11) larva of a robber fly (Diptera: Asilidae); (12) larva of a soldier fly (Dipt era: Stratiomyidae); (13) springtail (Collembola: Isotomidae); (14) larva of a weevil (Coleoptera: Curculionidae); (15) larva of a m uscid fly (Diptera: Muscidae); (16) proturan (Protura: Sinentomidae); (17) springtail (Collembola: Isotomidae); (18) larva of a March fly (Diptera: Bibionidae); (19) larva of a scarab beetle (Coleoptera: Scarabaeidae). (Individual organisms after various sources, especially Eisenbeis & Wichard 1987)
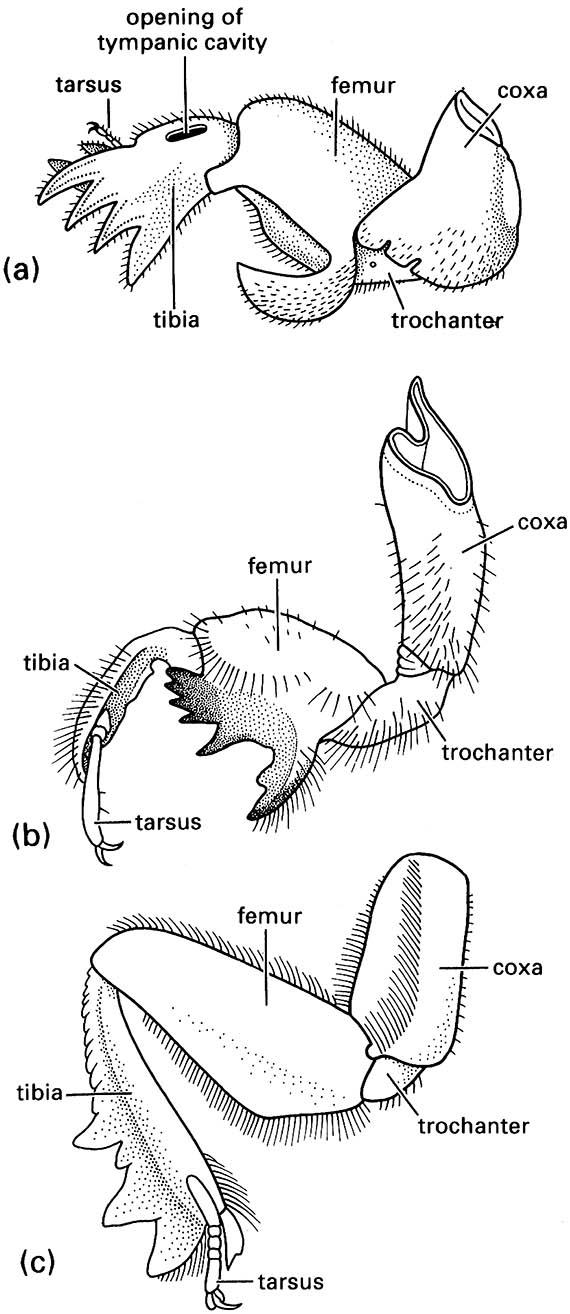
(a) a mole cricket of Gryllotalpa (Orthoptera: Gryllotalpidae); (b) a nymphal periodical cicada of Magicicada (Hemiptera: Cicadidae); and (c) a scarab beetle of Canthon (Coleoptera: Scarabaeidae). ((a) After Frost 1959; (b) after Snodgrass 1967; (c) after Richards & Davies 1977)
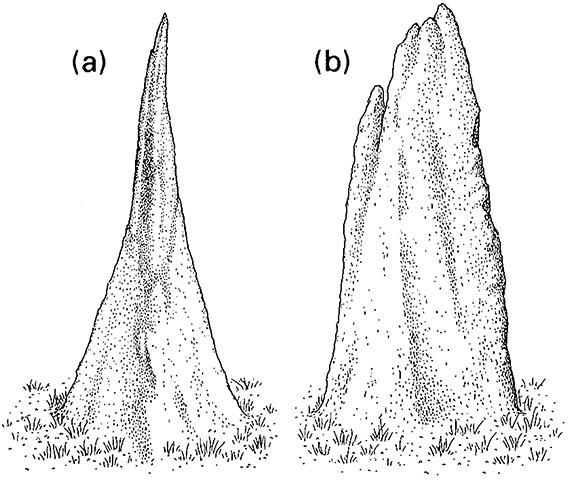
(a) the north-south view, and (b) the east-west view. (After Hadlington 1987)
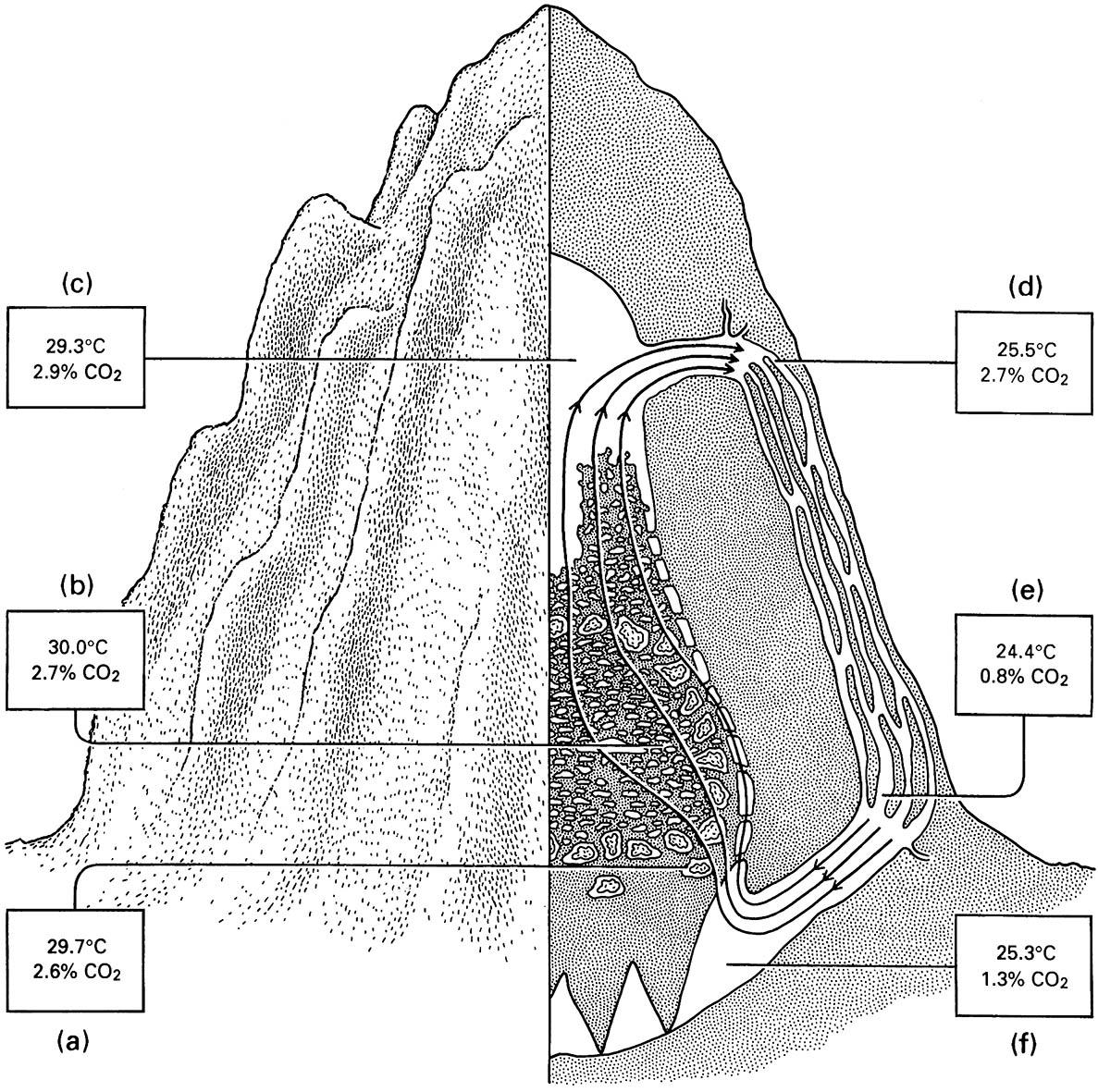
Measurements of temperature and carbon dioxide are shown in the boxes for the following locations: (a) the fungus combs; (b) the brood chambers; (c) the attic; (d) the upper part of a ridge channel; (e) the lower pa rt of a ridge channel; and (f ) the cellar. (After Lüscher 1961)