16.2.1. Insecticide resistance
Insecticide resistance is the result of selection of individuals that are predisposed genetically to survive an insecticide. Tolerance, the ability of an individual to survive an insecticide, implies nothing about the basis of survival. Over the past few decades more than 500 species of arthropod pests have developed resistance to one or more insecticides (Fig. 16.2).
The tobacco or silverleaf whitefly (Box 16.1), the Colorado potato beetle (Box 16.5), and the diamond-back moth (see discussion of Bt in section 16.5.2) are resistant to virtually all chemicals available for control. Chemically based pest control of these and many other pests may soon become virtually ineffectual because many show cross- or multiple resistance. Cross-resistance is the phenomenon of a resistance mechanism for one insecticide giving tolerance to another. Multiple resistance is the occurrence in a single insect population of more than one defense mechanism against a given compound. The difficulty of distinguishing cross-resistance from multiple resistance presents a major challenge to research on insecticide resistance. Mechanisms of insecticide resistance include:
- increased behavioral avoidance, as some insecticides, such as neem and pyrethroids, can repel insects;
- physiological changes, such as sequestration (deposition of toxic chemicals in specialized tissues), reduced cuticular permeability (penetration), or accelerated excretion;
- biochemical detoxification (called metabolic resistance) mediated by specialized enzymes;
- increased tolerance as a result of decreased sensitivity to the presence of the insecticide at its target site (called target-site resistance).
The tobacco budworm, Heliothis virescens (Lepidoptera: Noctuidae), a major pest of cotton in the USA, exhibits behavioral, penetration, metabolic, and target-site resistance. Phytophagous insects, especially polyphagous ones, frequently develop resistance more rapidly than their natural enemies. Polyphagous herbivores may be preadapted to evolve insecticide resistance because they have general detoxifying mechanisms for secondary compounds encountered among their host plants. Certainly, detoxification of insecticidal chemicals is the most common form of insecticide resistance. Furthermore, insects that chew plants or consume non-vascular cell contents appear to have a greater ability to evolve pesticide resistance compared with phloem- and xylem-feeding species. Resistance has developed also under field conditions in some arthropod natural enemies (e.g. some lacewings, parasitic wasps, and predatory mites), although few have been tested. Intraspecific variability in insecticide tolerances has been found among certain populations subjected to differing insecticide doses.
Insecticide resistance in the field is based on relatively few or single genes (monogenic resistance), i.e. owing to allelic variants at just one or two loci. Field applications of chemicals designed to kill all individuals lead to rapid evolution of resistance, because strong selection favors novel variants such as a very rare allele for resistance present at a single locus. In contrast, laboratory selection often is weaker, producing polygenic resistance. Single-gene insecticide resistance could be due also to the very specific modes of action of certain insecticides, which allow small changes at the target site to confer resistance.
Management of insecticide resistance requires a pro- gram of controlled use of chemicals with the primary goals of: (i) avoiding or (ii) slowing the development of resistance in pest populations; (iii) causing resistant populations to revert to more susceptible levels; and/or (iv) fostering resistance in selected natural enemies. The tactics for resistance management can involve maintaining reservoirs of susceptible pest insects (either in refuges or by immigration from untreated areas) to promote dilution of any resistant genes, varying the dose or frequency of insecticide applications, using less-persistent chemicals, and/or applying insecticides as a rotation or sequence of different chemicals or as a mixture. The optimal strategy for retarding the evolution of resistance is to use insecticides only when control by natural enemies fails to curtail economic damage. Furthermore, resistance monitoring should be an integral component of management, as it allows the anticipation of problems and assessment of the effectiveness of operational management tactics.
Recognition of the problems discussed above, cost of insecticides, and also a strong consumer reaction to environmentally damaging agronomic practices and chemical contamination of produce have led to the current development of alternative pest control methods. In some countries and for certain crops, chemical controls increasingly are being integrated with, and sometimes replaced by, other methods.
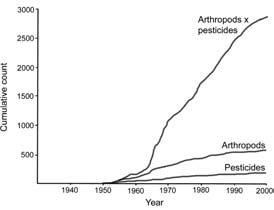
(After Bills et al. 2000)