10.3.4. Oxygen uptake with an open spiracular system
For aquatic insects with open spiracular systems, there is a range of possibilities for obtaining oxygen. Many immature stages of Diptera can obtain atmospheric oxygen by suspending themselves from the water meniscus, in the manner of a mosquito larva and pupa (Fig. 10.2). There are direct connections between the atmosphere and the spiracles in the terminal respiratory siphon of the larva, and in the thoracic respiratory organ of the pupa. Any insect that uses atmospheric oxygen is independent of low dissolved oxygen levels, such as occur in rank or stagnant waters. This independence from dissolved oxygen is particularly prevalent amongst larvae of flies, such as ephydrids, one species of which can live in oil-tar ponds, and certain pollution-tolerant hover flies (Syrphidae), the “rat- tailed maggots”.
Several other larval Diptera and psephenid beetles have cuticular modifications surrounding the spiracular openings, which function as gills, to allow an increase in the extraction rate of dissolved oxygen without spiracular contact with the atmosphere. An unusual source of oxygen is the air stored in roots and stems of aquatic macrophytes. Aquatic insects including the immature stages of some mosquitoes, hover flies, and Donacia, a genus of chrysomelid beetles, can use this source. In Mansonia mosquitoes, the spiracle-bearing larval respiratory siphon and pupal thoracic respiratory organ both are modified for piercing plants.
Temporary air stores (compressible gills) are common means of storing and extracting oxygen. Many adult dytiscid, gyrinid, helodid, hydraenid, and hydrophilid beetles, and both nymphs and adults of many belostomatid, corixid, naucorid, and pleid hemipterans use this method of enhancing gaseous exchange. The gill is a bubble of stored air, in contact with the spiracles by various means, including subelytral retention in adephagan water beetles (Fig. 10.3), and fringes of specialized hydrofuge hairs on the body and legs, as in some polyphagan water beetles. When the insect dives from the surface, air is trapped in a bubble in which all gases start at atmospheric equilibrium. As the submerged insect respires, oxygen is used up and the carbon dioxide produced is lost due to its high solubility in water. Within the bubble, as the partial pressure of oxygen drops, more diffuses in from solution in water but not rapidly enough to prevent continued depletion in the bubble. Meanwhile, as the proportion of nitrogen in the bubble increases, it diffuses outwards, causing diminution in the size of the bubble. This contraction in size gives rise to the term “compressible gill”. When the bubble has become too small, it is replenished by the insect returning to the surface.
The longevity of the bubble depends upon the relative rates of consumption of oxygen and of gaseous diffusion between the bubble and the surrounding water. A maximum of eight times more oxygen can be supplied from the compressible gill than was in the original bubble. However, the available oxygen varies according to the amount of exposed surface area of the bubble and the prevailing water temperature. At low temperatures the metabolic rate is lower, more gases remain dissolved in water, and the gill is long lasting. Conversely, at higher temperatures metabolism is higher, less gas is dissolved, and the gill is less effective.
A further modification of the air-bubble gill, the plastron, allows some insects to use permanent air stores, termed an “incompressible gill”. Water is held away from the body surface by hydrofuge hairs or a cuticular mesh, leaving a permanent gas layer in contact with the spiracles. Most of the gas is relatively insoluble nitrogen but, in response to metabolic use of oxygen, a gradient is set up and oxygen diffuses from water into the plastron. Most insects with such a gill are relatively sedentary, as the gill is not very effective in responding to high oxygen demand. Adults of some curculionid, dryopid, elmid, hydraenid, and hydrophilid beetles, nymphs and adults of naucorid bugs, and pyralid moth larvae use this mode of oxygen extraction.
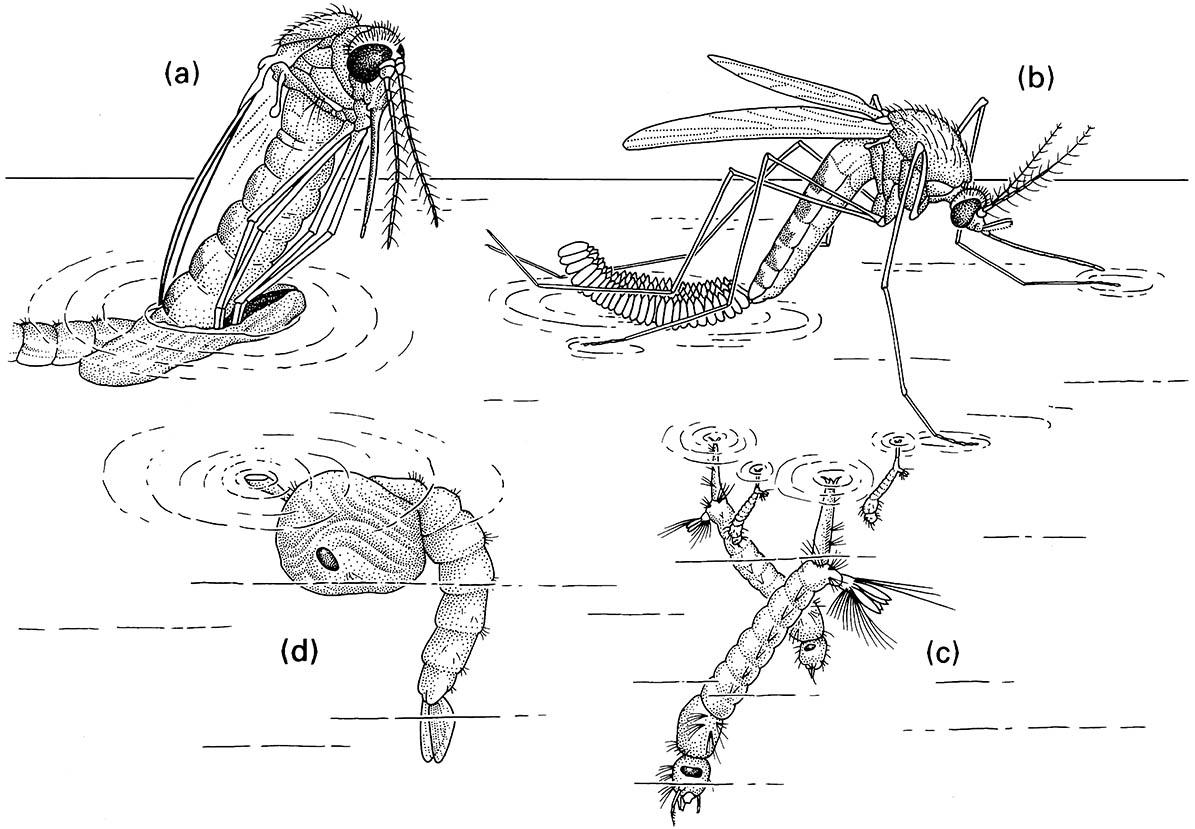
(a) adult emerging from its pupal exuviae at the water surface; (b) adult female ovipositing, with her eggs adhering together as a floating raft; (c) larvae obtaining oxygen at the wa ter surface via their siphons; (d) pupa suspended from the water meniscus, with its respiratory horn in contact with the atmosphere. (After Clements 1992)
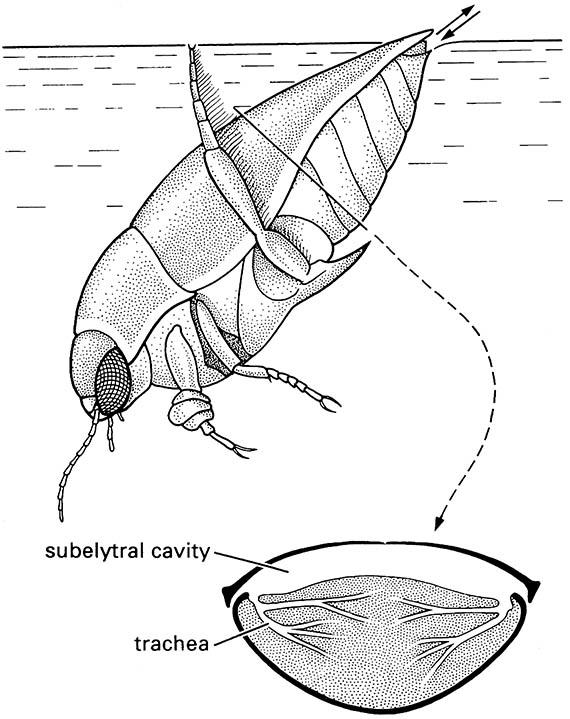
Below is a transverse section of the beetle’s abdomen showing the large air store below the elytra and the tracheae opening into this air space. Note: the tarsi of the fore legs are dilated to form adhesive pads that are used to hold the female during copulation. (Inset after Wigglesworth 1964)